EXTENSION OF TWO-WAY SATELLITE TIME- AND FREQUENCY TRANSFER METHOD TO REAL-TIME OPERATION AND CARRIER PHASE MEASUREMENTS
Wolfgang SCHÄFER, Alexander PAWLITZKI, Torge KUHN TimeTech GmbH,
Nobelstrasse 15, D-70569 Stuttgart, Germany
ABSTRACT
So far, Two-Way Satellite Time and Frequency Transfer (TWSTFT) was mainly used by primary laboratories to compare national time-scales. New concepts make such highly accurate methods available to the less sophisticated user at similar performance levels and in real-time operation. A method called .Two-Way Time Synchronisation via Satellite. is introduced, which provides low phase-noise time- and frequency signals synchronised to a remote reference clock. Results show an ADEV of 1E-14 for t > 104 s.
A frequency transfer method via satellite based on carrier phase measurements is presented. Dual receiver- channel modems are used to eliminate errors due to the satellite.s local oscillator and due to the satellite.s movement.
Initial experiments with two modems indicated the potential to reach a frequency transfer accuracy of 2*10- 15 m within 5 inutes. Although the concept of carrier phase was verified by test, these data could not yet be achieved with actual Very Small Aperture Terminal (VSAT) ground stations.
Different 2-way operational modes have been tested and verified during a 10 day test campaign. Carrier phase measurements are compared to simultaneous TWSTFT data to prove the concept. Carrier phase data exhibit good short term stability at the level of ADEV 1.2E-12 / sqrt(t) for t < 100 s.
The long-term stability however is in the same order of magnitude as TWSTFT data. This indicates, that significant improvements to RF signal generation and frequency distribution elements are still necessary to achieve the full potential of carrier phase data.
Tests have been performed with 10 MChip/s pseudo- noise coded signals via a free transponder provided by Societé Européene des Satellites, Luxembourg (SES).
1. INTRODUCTION
TWSTFT has been successfully in use to compare primary clocks in time-laboratories in the U.S., Europe and Far-East since more than 25 years. The method has the unique property to cancel most of the uncertainties of the radio signal propagation path at the cost of requiring rather elaborate transmit- and receive equipment at both sides of the link [1]. Despite it.s unique features, available satellite time and associated costs for this active service limited so far the use of the TWSTFT method to a few laboratories.
New operational features and modes of the SATRE (Satellite Time and Ranging Equipment) [2] modem have been developed in the past six years. Together with advanced satellite- and ground-station concepts being introduced by satellite operators, the TWSTFT method and it.s derived functions might become an attractive solution for a larger community exhibiting high performance at competitive costs. This would provide new opportunities for National Standard Laboratories to offer time- and frequency via satellite to commercial users.
This contribution intentionally does not cover such equally important items as ground station calibration and ground station delay monitoring. Extensive literature exists on these aspects. [3]
2. OPERATIONAL FEATURES
SATRE modems are designed to reduce necessary external hardware and test equipment. Delay measure- ments are performed internally. SATRE features automated operation, on-line data evaluation and full remote monitoring and control via serial link or via an IP network. There is a real-time data transmit capability via the satellite link to exchange Two-Way data and other corrective data in between partner stations. A built-in GPS receiver is available to determine the ground station location for automatic Sagnac correction.
The instrument accommodates up to three receiver channels or two transmit- and two receive channels.
Multi-link applications and simultaneous ground-station delay monitoring become feasible.
3. PERFORMANCE ASPECTS
Several aspects to improve instrument performance have been considered during the development. There are user selectable chip-rates ranging from 0.5 MChip/s to 20 MChip/s. High chip-rates not only reduce measurement noise, which could be overcome by longer observation periods, but achievable system accuracy is directly related to the chip-rate as well. This effect had been observed empirically in various projects.
Analytical work recently performed at NIST [4] clearly shows the advantages of higher chipping rates. The effect is mainly related to multipath- and changing multipath conditions inside the ground station and inside the equipment itself. The effect limits achievable system accuracy, long-term stability and reproducibility in between sessions. An empirical estimate on system reproducibility is Reproducibility = (125 .. 300 ps) / Chip-Rate The chip-rate to be entered in MChip/s. To achieve the lower limit, high performance phase-stable cables are usually required. High return loss at the RF connections are key to minimise multipath-induced errors. Reproducibility includes systematic errors encountered from session to session, which might be considerably higher than instrument drift alone would suggest. For example, a 10 MChip/s system [5] usually exhibits a reproducibility of 20 - 30 ps. 20 MChip/s systems reach an even lower system noise floor. TX-RX delay calibrations reported by VSL [3] are likely to be improved at a similar ratio when higher chip-rates are used.
Careful control of the shape of the transmitted spectrum is essential to achieve reproducibility. It is believed, that .closing errors. [6] at least partially have to be attributed to this effect. It can be shown, that a non-symmetric RF spectrum, i.e. differences between the shape of lower- and upper sideband, causes significant code-phase changes in the receiver.s delay- lock loop. Transmitted spectrum symmetry is ensured by wideband transmit filters, by use of a highly linear phase-modulator and by baseband filtering of the modulation signal.
All modulation frequencies and signal carriers are generated coherently to the reference frequency. In the receive path, measurements of code- and carrier phase are strictly related to that reference as well, leading to a fully coherent system. This feature is essential for the carrier phase method described below.
4. REAL-TIME TWSTFT
The capability to exchange measurement data in real- time via RF link directly leads to the possibility to present TWSTFT data on-line and directly on screen.
The operator has full visibility on the status of the two clocks being compared. There are smoothed data using parabolic interpolation with time periods of up to 5 minutes. Single-shot data are available once per second. Although being more noisy, they are used to detect clock- or system failures with a latency of 4 s.
5. MONITORING OF REMOTE CLOCKS
Real-time TWSTFT data are available at both partner stations of a time link. Both users see the difference and rate between both clocks. This feature is available in single- and multi-channel models. Using a three-channel version, up to three remote clocks can be monitored with a single unit at the central site. The local area network connection provides such data to remotely located users. A user might install its reference site with direct access to a highly stable reference clock and he can then observe the results remotely via an IP network.
Clock data are contained in the RF data stream. They can be received by any station within the footprint area of the satellite.
6. TWO-WAY TIME SYNCHRONISATION
Traditional TWSTFT links have been installed by owners of highly accurate clocks. Most users are even national standard laboratories. The term .transfer. might lead to the assumption, that this method requires availability of high stability clocks or primary references at both sites.
Fig 6-1: Two-Way Time Synchronisation
For such users not in the possession of highly stable clocks, an operational mode called .Two-Way Time Synchronisation. has been introduced (Fig. 6-1). In this configuration, the Two-Way modem to be synchronised is equipped with a low-phase-noise BVA oscillator or with a Rb reference. This internal oscillator replaces the external laboratory reference. It is synchronised to the clock on the distant site of the Two-Way time link using the TWSTFT method to compare both clocks in real- time. As result, the built-in clock is physically controlled to exhibit zero time- and frequency offsets to the remote clock using a computer-based control algorithm. Instead of requiring laboratory reference inputs as in TWSTFT mode, the modem in Two-Way Time Synchronisation mode outputs physical time and frequency signals together with date&time information, which are directly synchronised to the reference clock.
The characteristics of the built-in clock are chosen to optimise system performance. For a continuously available time-link or for such time-links, where frequent contacts to the reference station are possible, BVA crystal clocks are used, as such oscillators provide excellent phase-noise characteristics. For such time links, where communication gaps longer than one hour might exist, an internal Rb reference oscillator is chosen to improve hold-over capability.
In general, the requirements on the internal clock performance can be reduced in the same manner, as the Two-Way time-link performance is increased. A link using very high chip-rates and at high availability reduces demands on the clock to be synchronised.
The Two-Way mode of the system allows to monitor the synchronised clock at both sites of the link. In particular, any imperfections of the control loop are directly measured with a system exhibiting TWSTFT performance and real-time capability, as long as the satellite link is available. This feature is used to obtain corrective data on the clock being synchronised. Such data are output to the user to further improve the physical time information. In the present implemen- tation, corrective data characterise the physical clock error to within several tens of ps with a latency of 6s.
This system has the advantage of being self- contained, i.e. no local reference is required. The user can directly use the low phase-noise frequency- and time signals. The actual system reaches a 1 ns timing error within approx. 1-2 hours and reaches full frequency stability within several hours of operation. At both sites, the state of synchronism is available.
7. TWO-WAY CARRIER PHASE MEASUREMENTS
Carrier phase measurements have been successfully used in satellite tracking systems like NASA.s Deep Space Network. Carrier phase data provide significantly better precision under similar RF link conditions. The improvement to code-phase data is approximately equal to the ratio between carrier frequency and code-rate.
Improvements of 100 to 1000 could be expected using typical TWSTFT systems operating in Ku-band.
Traditional carrier phase systems use coherent transponders, whereas transponders onboard commercial communication satellites simply convert the input carrier with a non-coherent local oscillator.
Carrier phase information between transponder input and output is lost in this process. It can be shown, that under the assumption of an unknown local-oscillator frequency and using a satellite exhibiting equal relative velocity to both ground-stations, normal Two-Way formulas would suffice to remove all unknowns.
ESA PRARE/ERS-2 and the PRARE/Meteor-3
Space experience has been gained during ESA PRARE/ERS-2 and the PRARE/Meteor-3 project. PRARE is a high performance microwave experiment for geodetic science.
Within these contracts TimeTech was subcontractor and responsible for the following activities:
- Preparation of manufacturing and test documentation
- Manufacturing of on-board flight hardware by staff, certified to ESA or MIL standards
- Manufacturing of antennas
- Sub assembly tests
- System integration and all AIT/AIV activities
- Launch support
- On-site system calibration
TimeTech‘s further tasks within the PRARE Project:
- Automated test and data analysis system for the PRARE experiment
- PRARE reference time unit installed at DLR / D-PAF
- Also the proton radiation tests with the PRARE engineering model in Villigen, CH attained important knowledge to the company.
Fig 7-1: Two Way Carrier Phase Measurements
Actual satellite links exhibit significant un-symmetry of the relative velocities at the level of several m/s. This results in two unknown Doppler shifts at a level significantly larger than typical clock errors to be measured. A compensation scheme has been developed to solve for the 2 unknowns: (1) difference between relative ground station velocities and (2) ground clock frequency difference.
The set-up uses a 4-link configuration, where each ground station receives the signal from the remote site and its own signal via the same transponder (Fig 7-1).
The correct operation of the system has been verified by direct comparison between carrier-phase data and TWSTFT data from parallel test runs.
8. TEST CAMPAIGN AND RESULTS
A test campaign to verify function and performance of the different operational modes has been conducted.
10 MChip/s PN-modulated signals on Ku-band have been used. A free transponder with 30 MHz bandwidth was provided at no charge by SES. Four similar ground stations at Physikalisch Technische Bundesanstalt (PTB), Deutsches Zentrum für Luft- und Raumfahrt (DLR, near Munich), SES and TimeTech have been used (Fig 8-1). PTB provided time and frequency from an active H-Maser. DLR is equipped with a passive H- Maser. The modem at PTB has three receive channels.
It can serve three links simultaneously using different Pseudo-Noise (PN) codes. The transponder was available continuously and transmissions were inter- rupted for configuration changes only.
Fig. 8-1: Test campaign configuration
8.1 Two-Way Time Transfer
Modems at PTB and DLR were directly connected to the external H-maser references. ADEV from raw data at 1 s intervals is shown in Fig 8.1-1.
Fig 8.1-1: Two-Way Time Transfer, 125000s, ADEV
TDEV is shown in Fig 8.1-2. These data are obtained from the built-in delay measurement system.
Fig 8.1-2: Two-Way Time Transfer, 12500s, TDEV
Fig 8.1-3: Two-Way Time Transfer, Phase
8.2 Two-Way Time Synchronisation
The 3-channel modem at PTB was connected to PTB.s H-Maser reference. The modem at DLR used its internal BVA oscillator (Oscilloquartz 8607), being synchronised to the PTB signals. A phase comparator (TimeTech, ADEV approx. 3E-12 / ) measures the modem.s 10 MHz output with reference to DLR.s H- maser. A time-interval counter (Stanford Research SR520) was used in parallel to verify un-ambiguous operation and to verify the 1pps output of the modem with respect to DLR.s H-Maser. The test set-up is shown in Fig. 8.2-1. The test lasted 240 000s.
Fig 8.2-1: Test of Two-Way Time Synchronisation
The modem uses its inherent TWSTFT function to continuously monitor the synchronisation state of the built-in BVA oscillator. These data are used to calculate a corrective term as estimate of the actual error of the physical output sgnals. The resulting ADEV and TDEV is shown in Figs. 8.2-2 and 8.2.-3. TDEV are shown in Fig 8.3-3 and Fig. 8.3-4. The good short term stability is clearly visible, however, the long- term stability of phase data only matched those obtained from TWSTFT results.
Fig 8.3-2: Carrier Phase Data, drift of clocks
8.2 Two-Way Time Synchronisation
Fig 8.3-3: Carrier Phase Data, 64000s, ADEV
Fig 8.3-4: Carrier Phase Data, 64000s, TDEV
8.4 Monitoring of remote clocks
The installation at PTB was used to monitor two remote clocks: One BVA oscillator in Two-Way Time Synchronisation mode at DLR and one GPS-disciplined Rb oscillator (GPS: Motorola, Rb: Tekelec Time, algorithm: TimeTech) at SES (Fig 8.4-1).
Fig 8.4-1: Two Remotely Monitored Clocks
The performance gain of Two-Way Time Synchroni- sation with respect to a GPS-based system is clearly visible.
9. CONCLUSIONS
Advanced operational modes for Time Transfer via Satellite have been introduced and verified by test.
Carrier phase measurements show good short term stability. Long term stability is so far limited by hardware and software imperfections. Improvements are certainly possible with dedicated ground station hardware.
Two-Way Time Synchronisation is a very promising mode for users without access to precise clocks. Short term stability exceeds the TWSTFT mode due to fly- wheel characteristic of the built-in BVA-type oscillator.
Otherwise, the results compare favourably to TWSTFT data. This makes the method an attractive solution to provide UTC-traceable time-signals available at full performance with a latency of 6 s only. Low-cost ground terminals used for return channel applications are being introduced, which could make this mode a cost effective solution.
10. ACKNOWLEDGEMENTS
Provision of precise time references by PTB and DLR were key to achieve precise results. The tests could not have been conducted without contribution of trans- ponder time free of charge by SES. The invaluable and continuous support by the three institutions is greatly appreciated.
11. REFERENCES
[1] Kirchner, D. (1991), Two-Way Time Transfer via Communication Satellites, Proc. IEEE 79(7), pp. 983ff
[2] Dienert, M., Baumer, C. (1993), High Accuracy Time Transfer and Ranging Experiments using SATRE Modems, Proc. of the 7th EFTF, March 16- 18, Neuchâtel, Switzerland, pp. 345ff
[3] de Jong, G. (1998), Delay Stability of the TWSTFT Earth Station at VSL, Proc. of the 12th EFTF, March 10-12, Warsaw, Poland, pp. 175ff
[4] Ascarrunz, F.G. et al (1999), Pseudo-Random Code Correlator Timing Errors due to Multipath, in these Proceedings
[5] Bedrich, S. (1998), Hochgenaue satellitengestützte Zeitübertragung mit PRARE, Scientific Technical Report STR98/24, GeoForschungsZentrum Potsdam
[6] Davis, J. A. (1995), Closing Errors Observed during Two-Way Time Transfer Experiments, Proc. of the 9th EFTF, Besancon, France, pp. 81ff
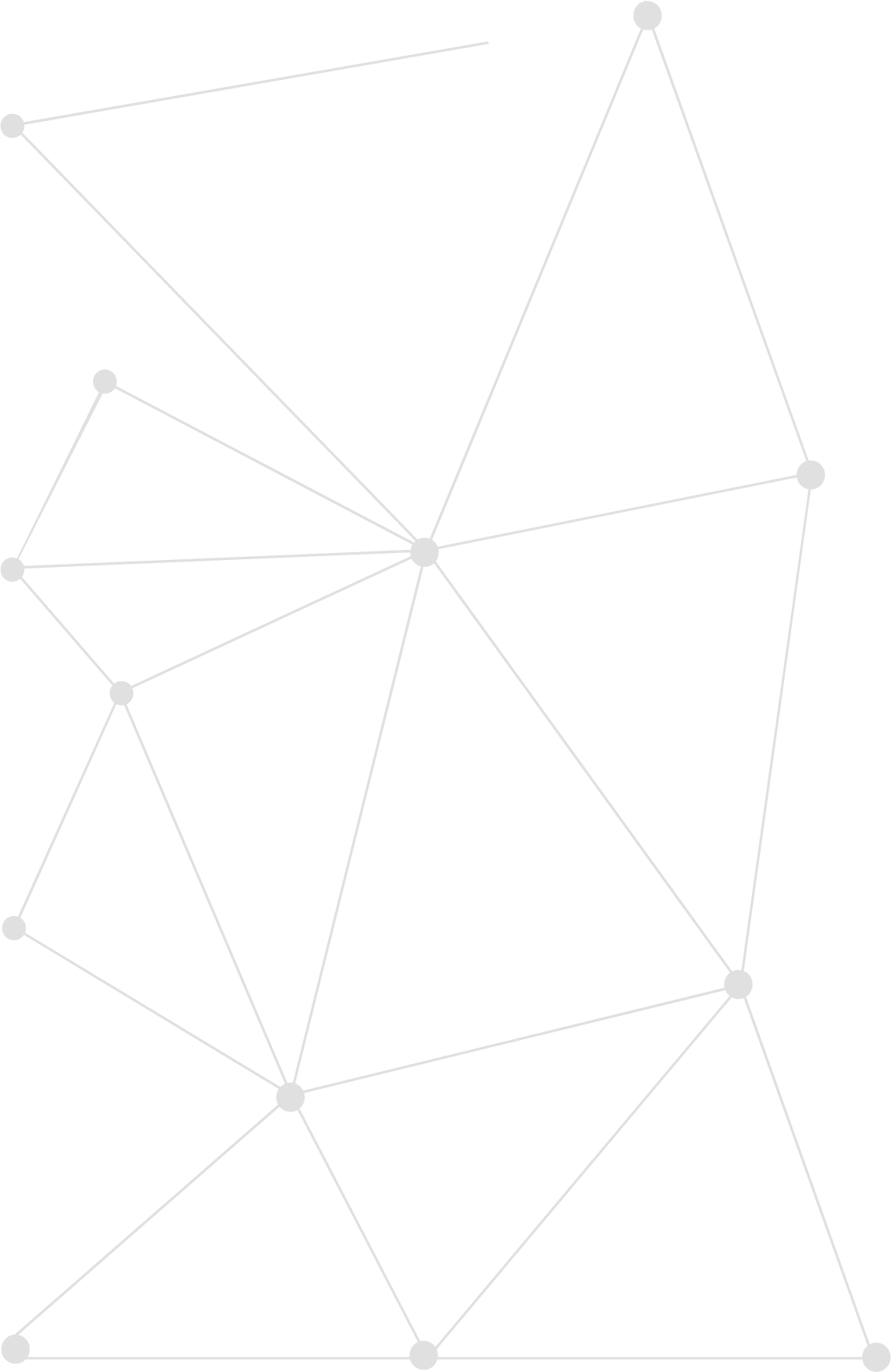
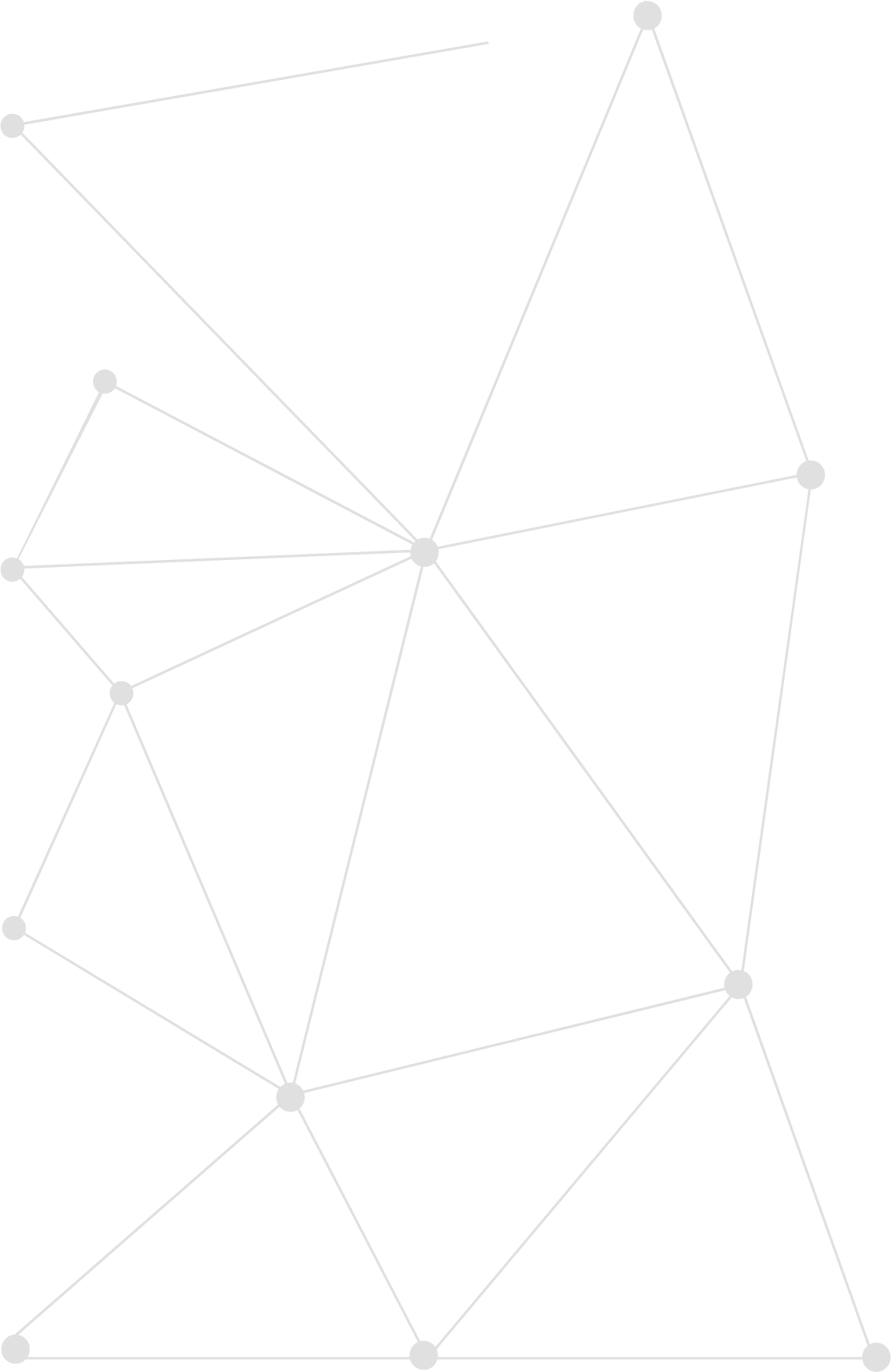